Design
Constructing microbial cell factories for monoterpene natural products
In this study, we chose nerol, borneol, and linalool as the desired target products in our cell factory. The mevalonate (MVA) pathway serves as the primary biosynthetic route leading to the formation of the monoterpene precursors geranyl diphosphate (GPP) or nerolyl pyrophosphate (NPP). Notably, the MVA pathway is endogenously present in the Y. lipolytica PO1f strain. However, PO1f lacks the inherent ability to naturally synthesize various secondary metabolites of monoterpenes. To overcome this limitation, we transfered an exogenous monoterpene synthase gene into the PO1f strain. Upon its expression, the precursor GPP/NPP, produced through the MVA pathway, undergoes enzymatic conversion to produce the above three target monoterpene products. The monoterpene synthases utilized in this investigation were selected based on their previously reported high and consistent microbial production yields.
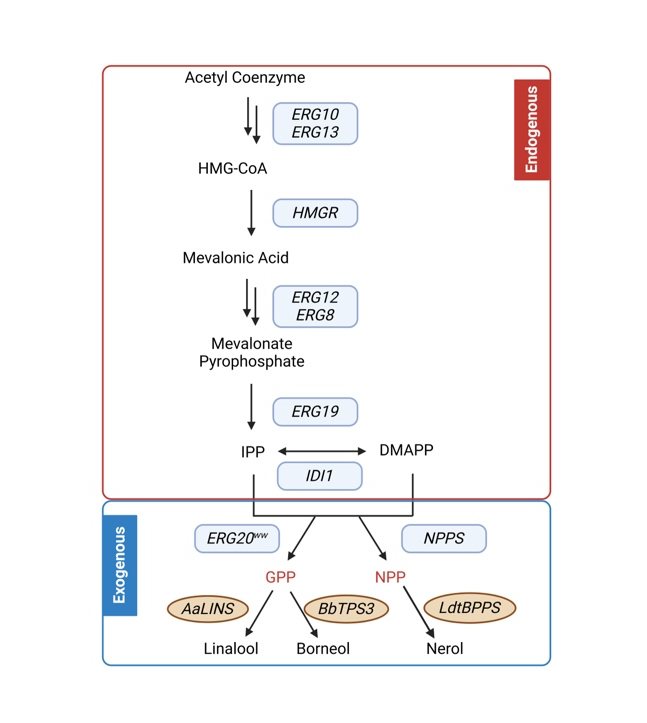
Fig.1. The MVA metabolic pathway and the metabolic pathways controlled by exogenous monoterpene synthases we introduced.
To overcome this limitation, we transfered an exogenous monoterpene synthase gene into the PO1f strain. Upon its expression, the precursor GPP/NPP, produced through the MVA pathway, undergoes enzymatic conversion to produce the above three target monoterpene products. The monoterpene synthases utilized in this investigation were selected based on their previously reported high and consistent microbial production yields.
Establishing circular monoterpene biosensor in Y. lipolytica
To construct a borneol biosensor in Y. lipolytica, it is imperative to express the borneol-induced reporter gene. In this study, we employed the allosteric transcription factor CamR manipulation system from Pseudomonas to heterologously express the inhibitory operon CamR in Y. lipolytica. The CamR binding sequence was introduced between the TATA box and the transcription start site (TSS) of the endogenous strong promoter pTEF1, thereby converting the promoter into a borneol-inducible promoter. As the reporter gene, we selected hrGFP, and the initiation efficiency of the promoter was evaluated by measuring the fluorescence intensity per OD600 (RFU/OD600).
In the absence of borneol, the CamR protein binds to the inserted sequence within the promoter, resulting in the inhibition of downstream hrGFP gene transcription. As a consequence, the yeast either does not emit any fluorescence or only exhibits weak fluorescence (off state). Conversely, when borneol is present in the environment, CamR binds to borneol and undergoes allosteric changes, preventing its interaction with the inserted sequence in the promoter. This relieves the inhibition on reporter gene expression. Consequently, the promoter initiates transcription, leading to the emission of green fluorescence by the yeast (on state).
The induction effect of borneol on reporter gene expression showed a positive correlation with the concentration of borneol within a specific range. Therefore, the concentration of borneol in the yeast culture could be accurately reflected by measuring the fluorescence intensity.
Due to the complex promoter structure of yeast, the insertion position of CamR binding sequence may have a great influence on the induction efficiency of the sensor. We scanned the insertion site of the 48 bp sequence between the TATA box and the transcription start site, and inserted the CamR binding sequence step by step with 6 bp length to form eight promoters with different CamR binding sequence insertion positions, and tested their induction efficiency at different borneol concentrations.
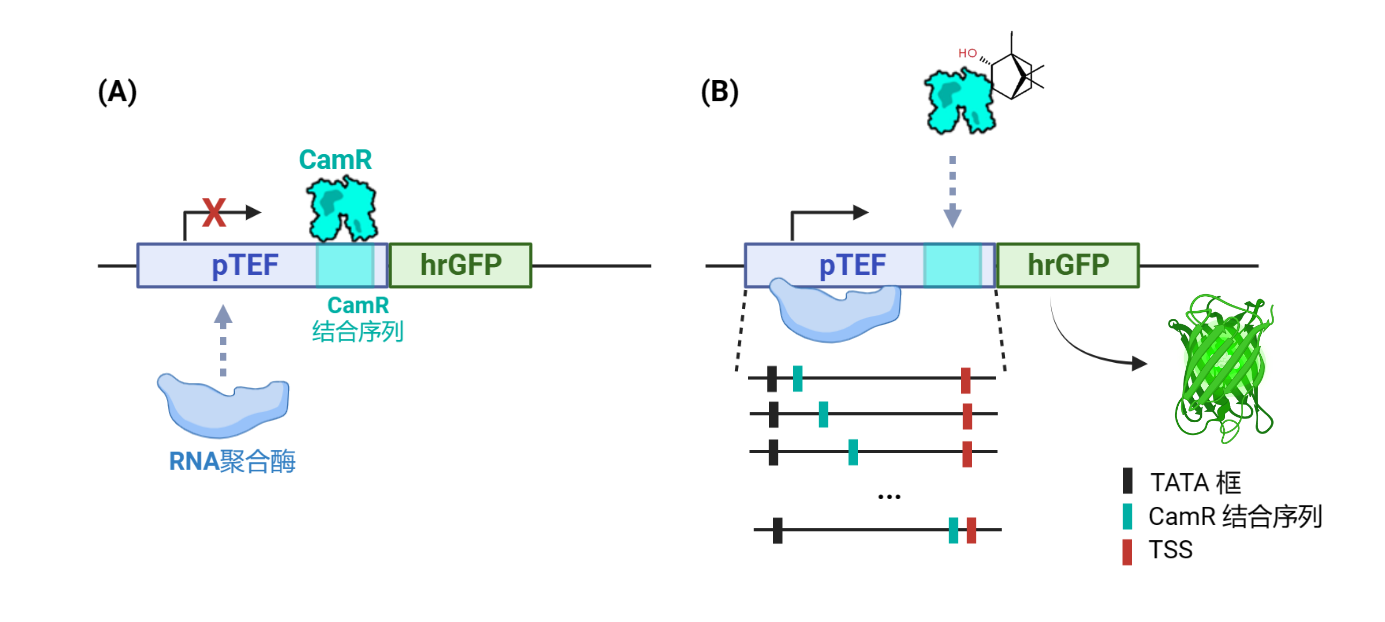
In the absence of borneol, the CamR protein binds to the inserted sequence within the promoter, resulting in the inhibition of downstream hrGFP gene transcription. As a consequence, the yeast either does not emit any fluorescence or only exhibits weak fluorescence (off state). Conversely, when borneol is present in the environment, CamR binds to borneol and undergoes allosteric changes, preventing its interaction with the inserted sequence in the promoter. This relieves the inhibition on reporter gene expression. Consequently, the promoter initiates transcription, leading to the emission of green fluorescence by the yeast (on state).
The induction effect of borneol on reporter gene expression showed a positive correlation with the concentration of borneol within a specific range. Therefore, the concentration of borneol in the yeast culture could be accurately reflected by measuring the fluorescence intensity.
Due to the complex promoter structure of yeast, the insertion position of CamR binding sequence may have a great influence on the induction efficiency of the sensor. We scanned the insertion site of the 48 bp sequence between the TATA box and the transcription start site, and inserted the CamR binding sequence step by step with 6 bp length to form eight promoters with different CamR binding sequence insertion positions, and tested their induction efficiency at different borneol concentrations.
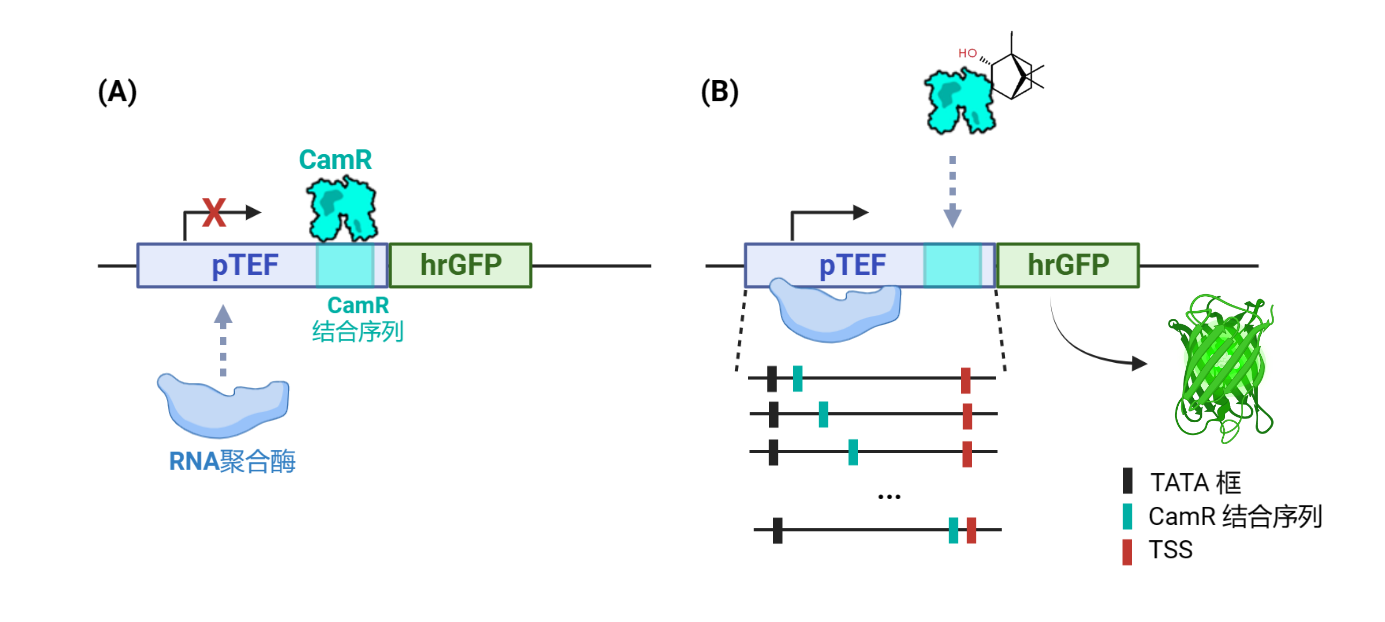
Fig.2. Two states of gene expression controlled by the repressor protein CamR. (A) Off state. (B) On state.
Building the synthetic pathway of Tagatose in K. marxianus
Synthesis of tagatose requires galactose as the precursor, and there are two common synthesizing pathways. One is L-arabinose isomerase (AI) directly converts D-galactose into D-tagatose, the other is xylose reductase (XR) reduces galactose into galactitol first, and then galactitol dehydrogenase (GDH) oxidizes galactitol into tagatose.
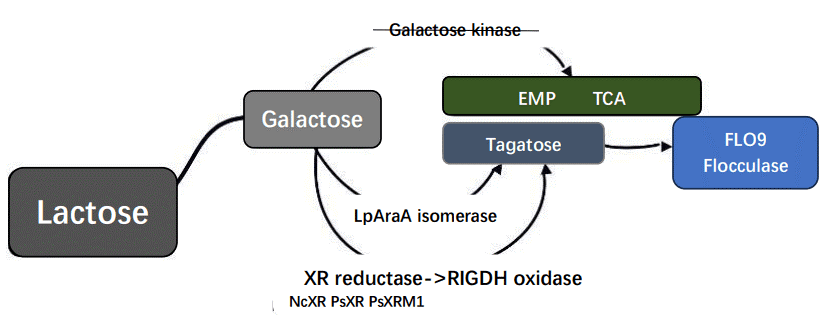
Fig.3. Two biological pathways for the metabolism of lactose in the production of tagatose.
Considering lab conditions, growing conditions, efficiency, time and so on, we utilized K. marxianus to be biological chassis to produce tagatose. And because of the endogeneity of β-galactosidase for K. marxianus, we chose lactose to be the substrate to reduce our costs. Besides, to accumulate galactose in cells and enhance utilization rate, we also designed the KnBox plasmid to knock out the gene coding for galactose kinase through homologous recombination, which catalyzes the first step of the Leloir pathway responsible for galactose assimilation in yeast.
Building a Tagatose biosensor in K. marxianus
During the research process We have learnt a gene cluster in E. coli Nissle 1917(EcN) that controls the metabolism of tagatose. Since the opening and closing of this gene cluster are induced by tagatose, we speculated that the cluster must contain a regulatory factor which can bind tagatose and regulate gene expression. Therefore, we attempted to transplant the entire gene cluster from EcN into the tagatose production platform in order to achieve efficient endogenous detection of tagatose. To achieve this, we ingeniously substituted the RS18695 gene within the gene cluster responsible for regulating the expression of aldolase, that can be efficiently complemented by the host's own enzymes. In its place, we introduced eGFP, a fluorescent protein. This strategic modification allowed us to construct the expression plasmid pTag, paving the way for subsequent experiment.
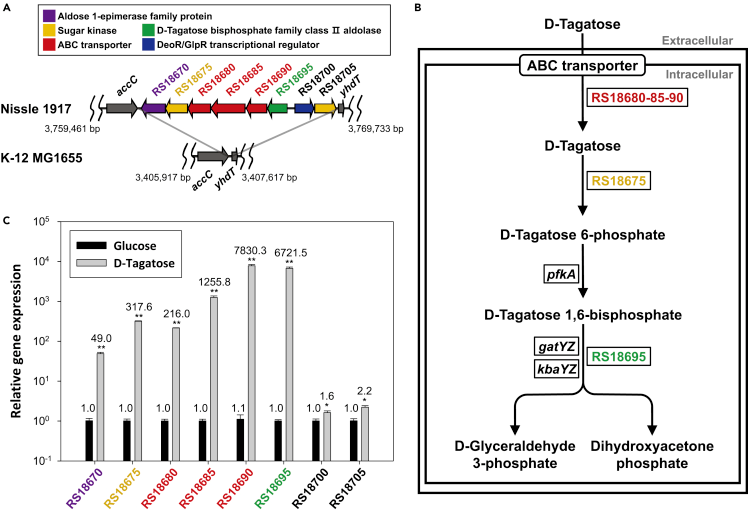
Fig.4. (A) Gene cluster for D-tagatose degradation in E. coli Nissle 1917 (EcN). (B) Proposed D-tagatose utilization pathway associated with EcN tag cluster. (C) Relative gene expression levels in EcN growing on D-tagatose or glucose. (cited from Ha,J.,Kim,D., iScience 2022)
Constructing and testing the nyEvolvR system in K. marxianus
To successfully express and apply the nyEvolvR system in K. marxianus, we constructed a shuttle vector named pKmEvolvR. This vector carries the sgRNA expression cassette, URA3 selection tag, and a fusion protein of enCas9 and PolI5M which is derived from E. coli's error-prone DNA Polymerase I. All the codons were specifically tailored to optimize for yeast. Additionally, considering the presence of a nucleus in eukaryotic cells, we incorporated multiple nuclear localization sequences (NLS), SV40, into the fusion protein. Furthermore, the efficiency of introducing mutations is directly influenced by the expression level of the fusion protein. Therefore, we utilized the high-expression pTEF1 promoter to drive the nyEvolvR system. Ultimately, we obtained a shuttle vector containing the nyEvolvR system targeting K. marxianus, which can be used for yeast transformation.
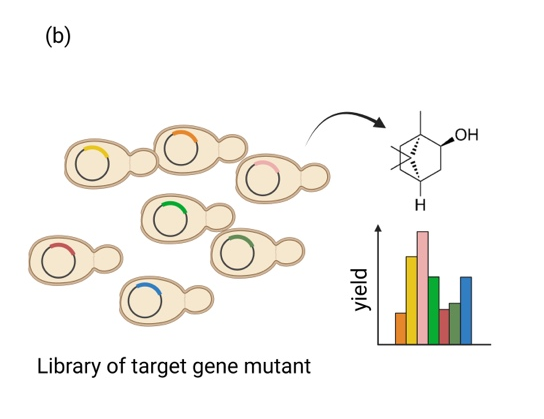
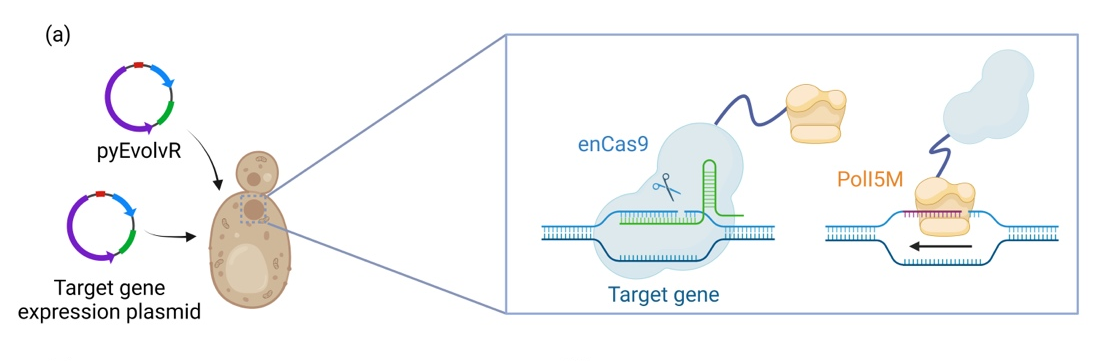
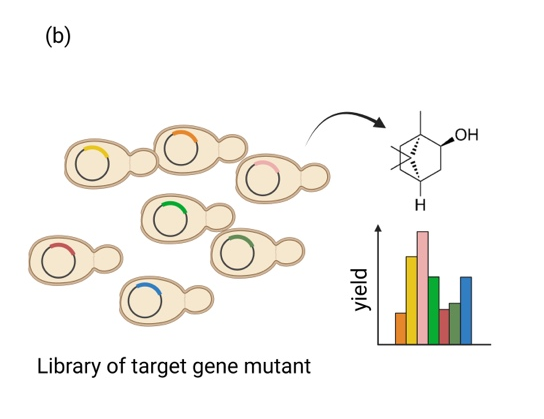
Fig.5. (a) Construction and directed evolution principles of the EvolvR system in nonconventional yeast. (b) Efficient detection of difficult-to-detect substances through biosensor.
Next :Results Page