In order to achieve de novo synthesis of HMA, we employed metabolic engineering and directed evolution strategies to investigate and address two key challenges, including insufficient precursor supply and limited activity of crucial enzymes, in the biosynthesis of 4-hydroxymandelate (HMA).
Through gene overexpression and multi-level gene interference using the CRISPRi approach, we successfully engineered an E. coli chassis strain capable of stably producing the essential substrate 4-hydroxyphenylpyruvate (HPP) and the titer reached 0.91 g/L. In a high-throughput screening process using a biosensor based on allosteric transcription factors, we eventually obtained a mutant clone, HmaSV152G, with a 125% improvement in the catalytic rate.
By combining directed evolution and metabolic engineering to improve enzyme activity and optimize the synthesis pathway, we successfully achieved de novo synthesis of HMA. In fermentation, we achieved the high production of 3.63 g/L HMA in 24 h with a single supply of 20 g/L glucose.
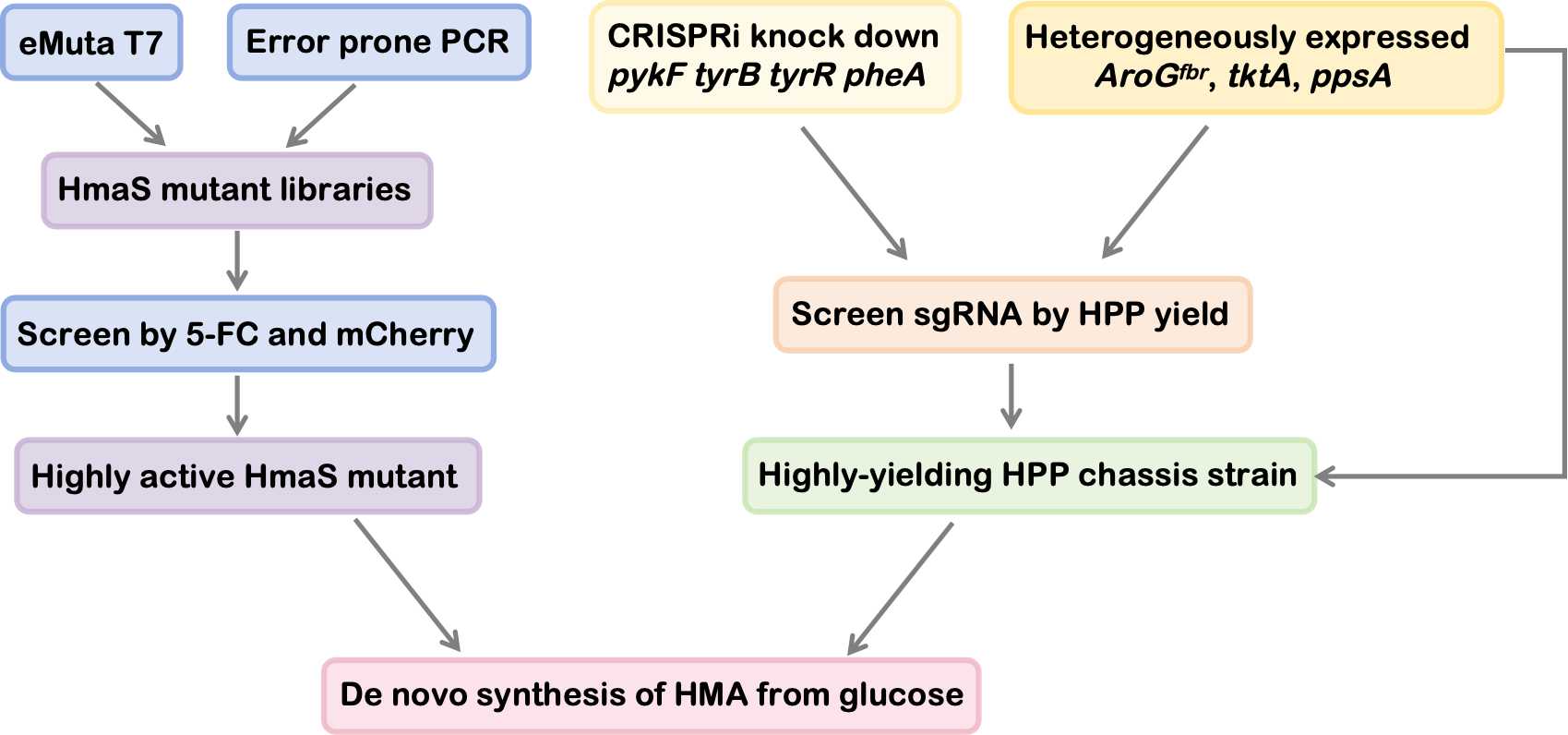
Molecular Evolution: HmaS
Construction of the hmaS Mutant Library
Random Mutant Strategy
We employed the approach of error-prone PCR, a discontinuous random mutagenesis method, by adjusting Mn2+and Mg2+ concentrations and ratio to manipulate the mutation rate. Simultaneously, we used a low-fidelity DNA polymerase increasing the possibility of mutation.
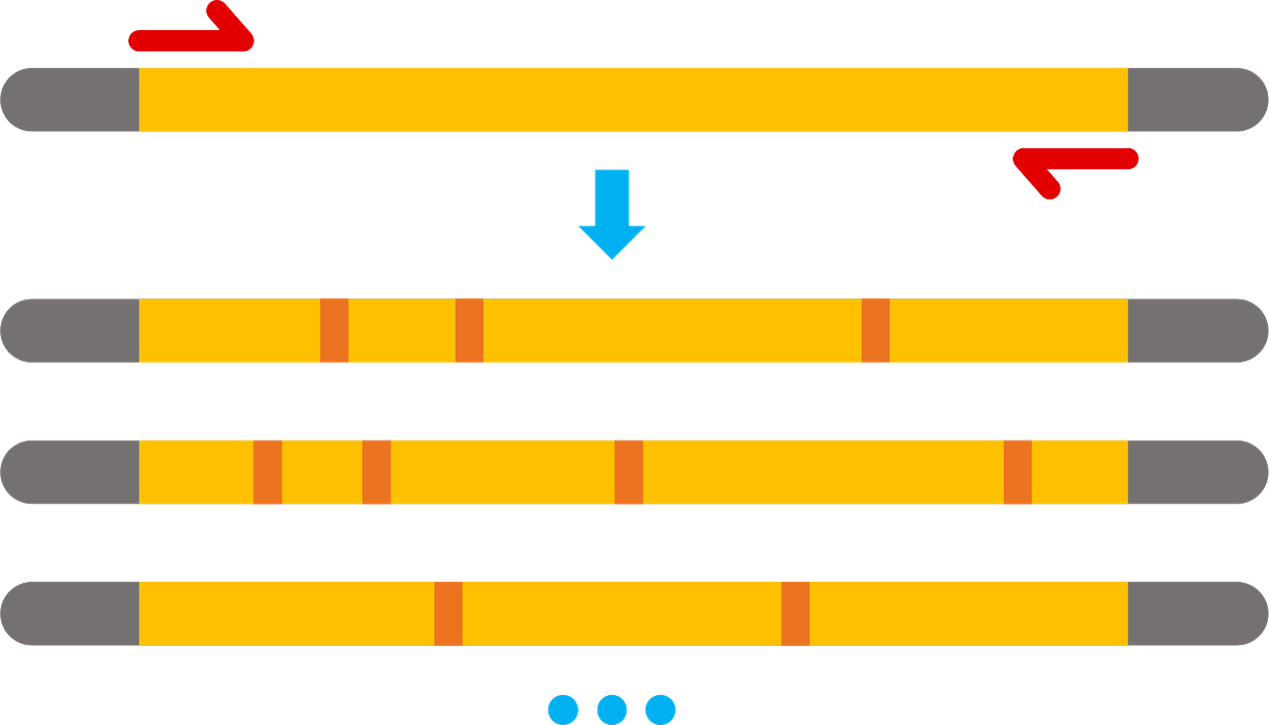
Continuous Mutant Strategy in vivo
In addition, we had constructed the eMuta T7 plasmid for expression in E. coli, which can express the fusion protein of T7 RNA polymerase and cytidine deaminase to generate mutations continuously. The high specificity of T7 RNA polymerase and T7 promoters enabled the specific mutations during transcription. The cytidine deaminase converted cytosine to uracil by deamination reaction on the exposed coding strand.
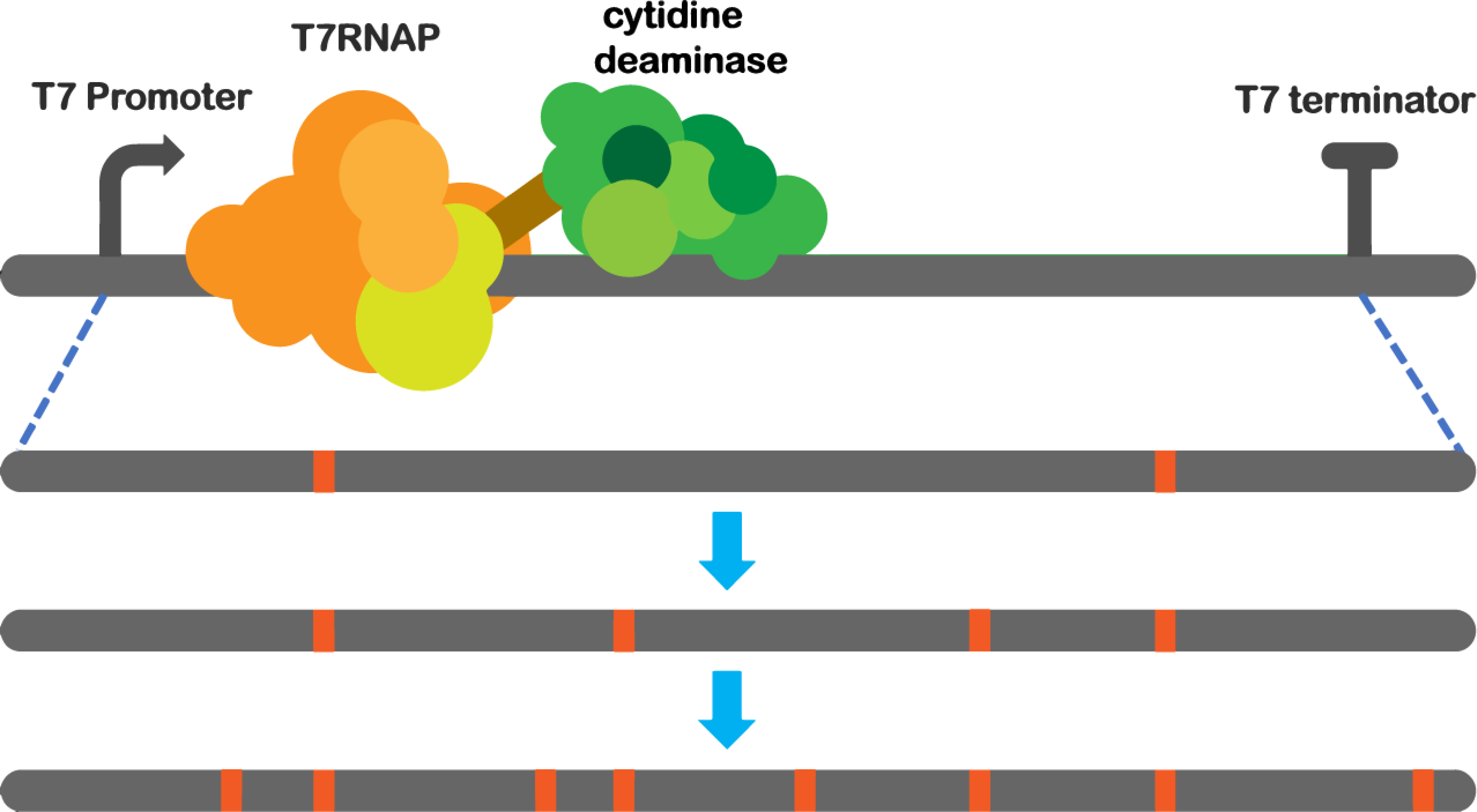
Dual-Module Screening Platform
In order to obtain highly active HmaS mutant individuals, we designed a screening plasmid containing two modules: the catalysis module and the sensing module. In the catalytic module, the hmaS gene is regulated by a constitutive promoter. The sensing module contains two important reporter genes: the red fluorescent protein mCherry gene and the cytosine deaminase codA gene that converts 5-fluorocytosine (5-FC) to 5-fluorouracil (5-FU), which kills bacteria. HPP levels decrease in response to high HmaS enzymatic activity, resulting in weak reporter signal, and the strain shows a light color and can survive in the medium containing 5-FC.
Pathway Evolution: Metabolic Engineering Modified HPP High-yield Chassis Strain
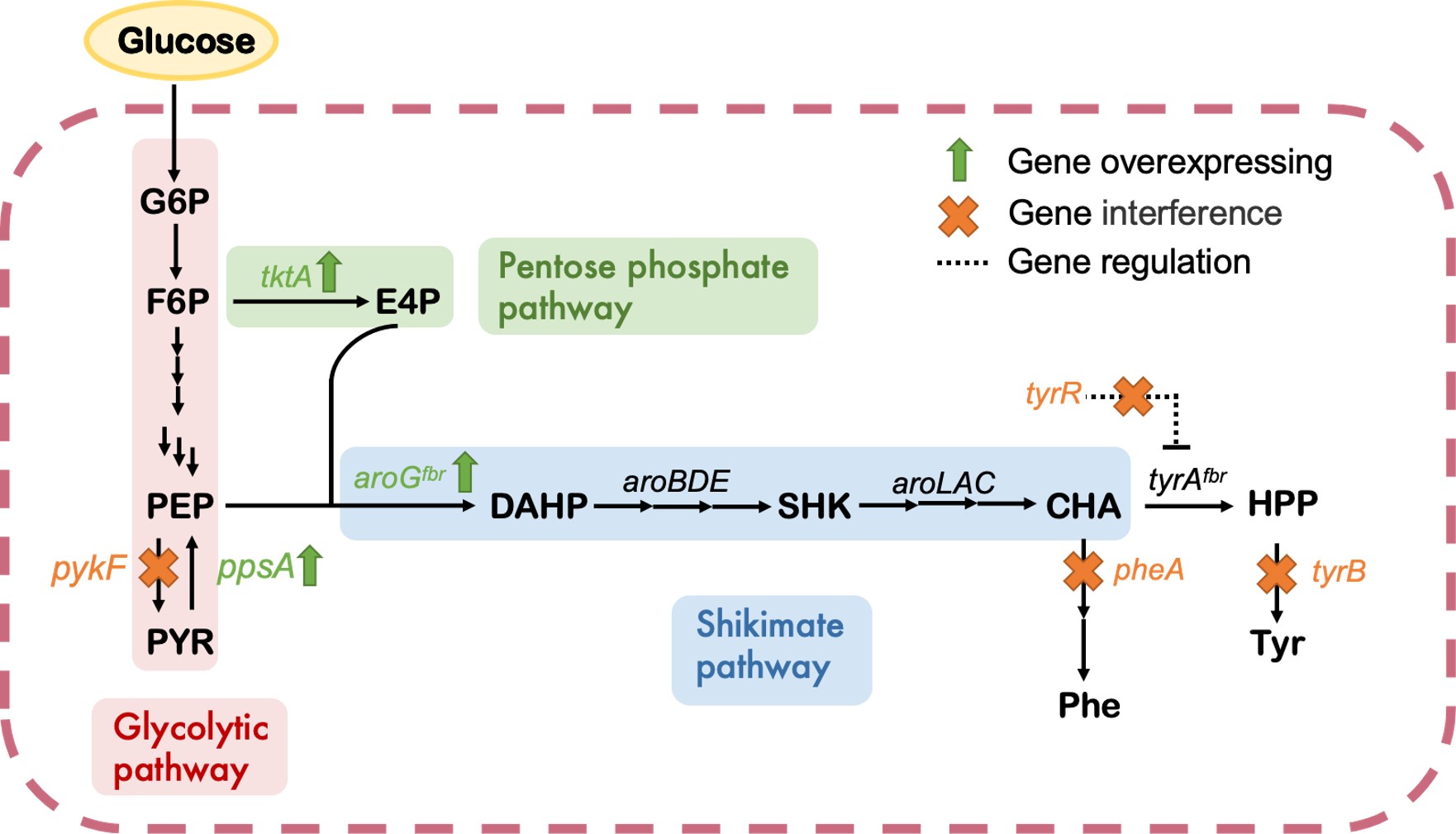
We aimed to utilize E. coli endogenous glycolytic pathway, pentose phosphate pathway and shikimate pathway, which can achieve de novo HPP synthesis with glucose as a substrate. However, under natural conditions, the accumulation of aromatic compounds in E. coli is limited, which prevents us from using HPP as a catalytic substrate. Therefore, we designed two strategies: overexpressing and silencing key genes in biosynthesis pathways, to improve the capability of bacteria for HPP synthesis.
Overexpression of Key Genes to Enhance the Precursor Supply
Phosphoenolpyruvate (PEP) and erythrose-4-phosphate (E4P) are two important precursor molecules for the synthesis of aromatic compounds. However, the fact of limited flux inhibits the synthesis of downstream products.
We constructed the pSB1c-aroGfbr-tktA-ppsA (pATP) plasmid, in which the expression of three key genes was controlled by an arabinose-inducible promoter. Overexpression of the phosphoenol pyruvate synthase (ppsA) gene reduced PEP flowing towards pyruvate, leading to PEP accumulation. To enhance E4P supply, we overexpressed the transketolase (tktA) gene to promote fructose-6-phosphate consumption. With overexpressed aroGfbr gene, both crucial precursor molecules showed flux into the shikimate pathway.
CRISPRi-mediated Gene Suppression to Reduce Competitive Pathway
In order to achieve multi-level inhibition of competitive genes for HPP production, we employed the CRISPRi technique based on mismatch sgRNAs. To alter the binding efficiency of the dCas9 protein to the target genes and generate various levels of inhibition, we introduced mutations in the 7-8 bp of sgRNA spacers to create mismatched sgRNAs, which totally encompassed 16 possible variants. Through assessing the impact of different sgRNA variants on HPP production, we successfully selected the optimal inhibition strength for the competitive genes, leading to enhanced HPP production and minimized growth stress caused by the gene knockout.
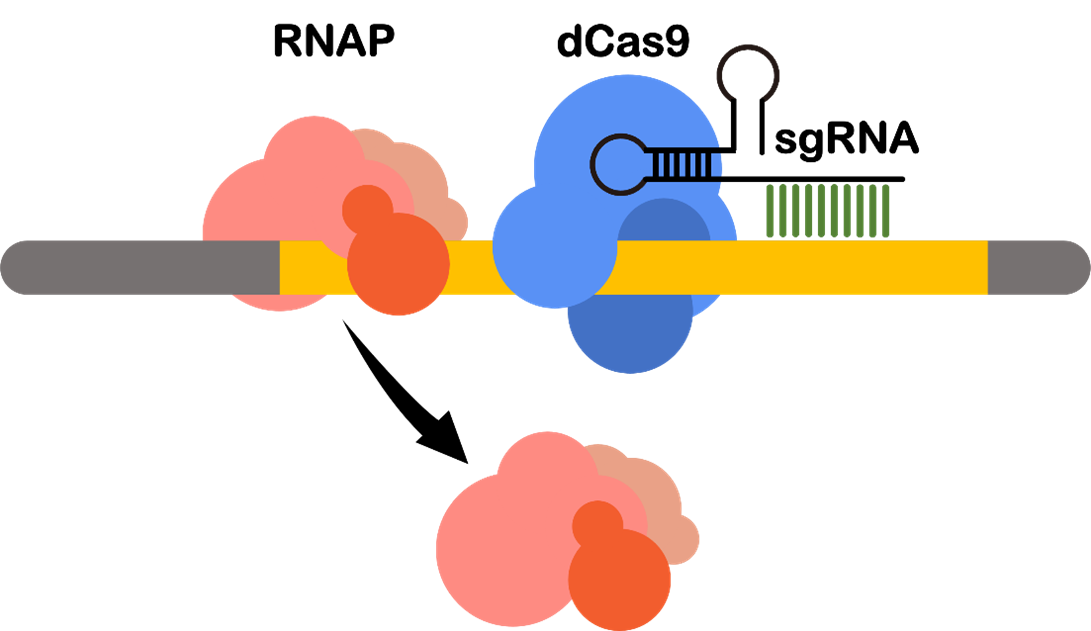
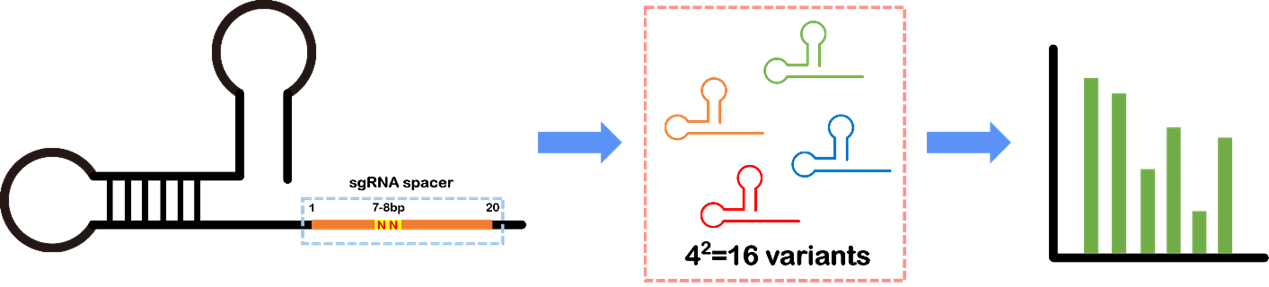
we selected 4 relevant genes pykF, tyrB, tyrR and pheA as targets of inhibition. These four genes are involved in the pathways leading to the synthesis of alanine, tyrosine and phenylalanine. To test for monogenic and polygenic inhibition, we designed corresponding mismatch sgRNA libraries for these four genes, and added BsaI restriction sites at both ends of all sgRNAs. By mixing four mismatch sgRNA libraries and a carrier plasmid containing dCas9 and other necessary parts, using the Golden Gate method to randomly assemble the four mismatch sgRNAs into one plasmid, we had access to a multiple mismatched sgRNAs library for polygenic inhibition.
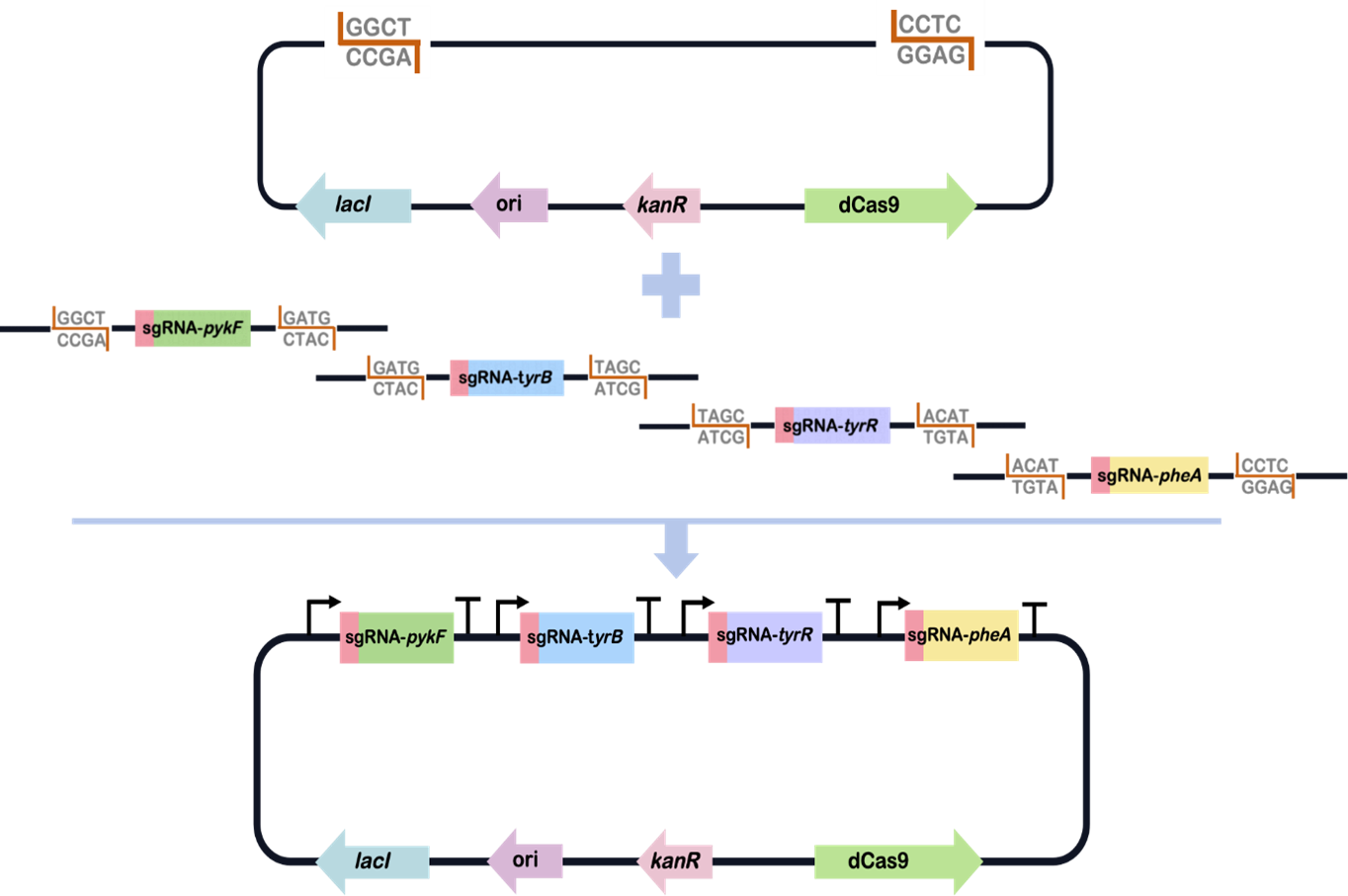
Modeling and Docking
To analyse the effects of amino acid substitutions on the response of HmaS protein and the interaction between small molecule ligands and the protein receptor, we used AlphaFold2 to simulate the protein structure of the PobR mutant and Autodock Vina to simulate the docking of HPP to the protein. The results of the docking were visualized by Pymol and LigPlus.
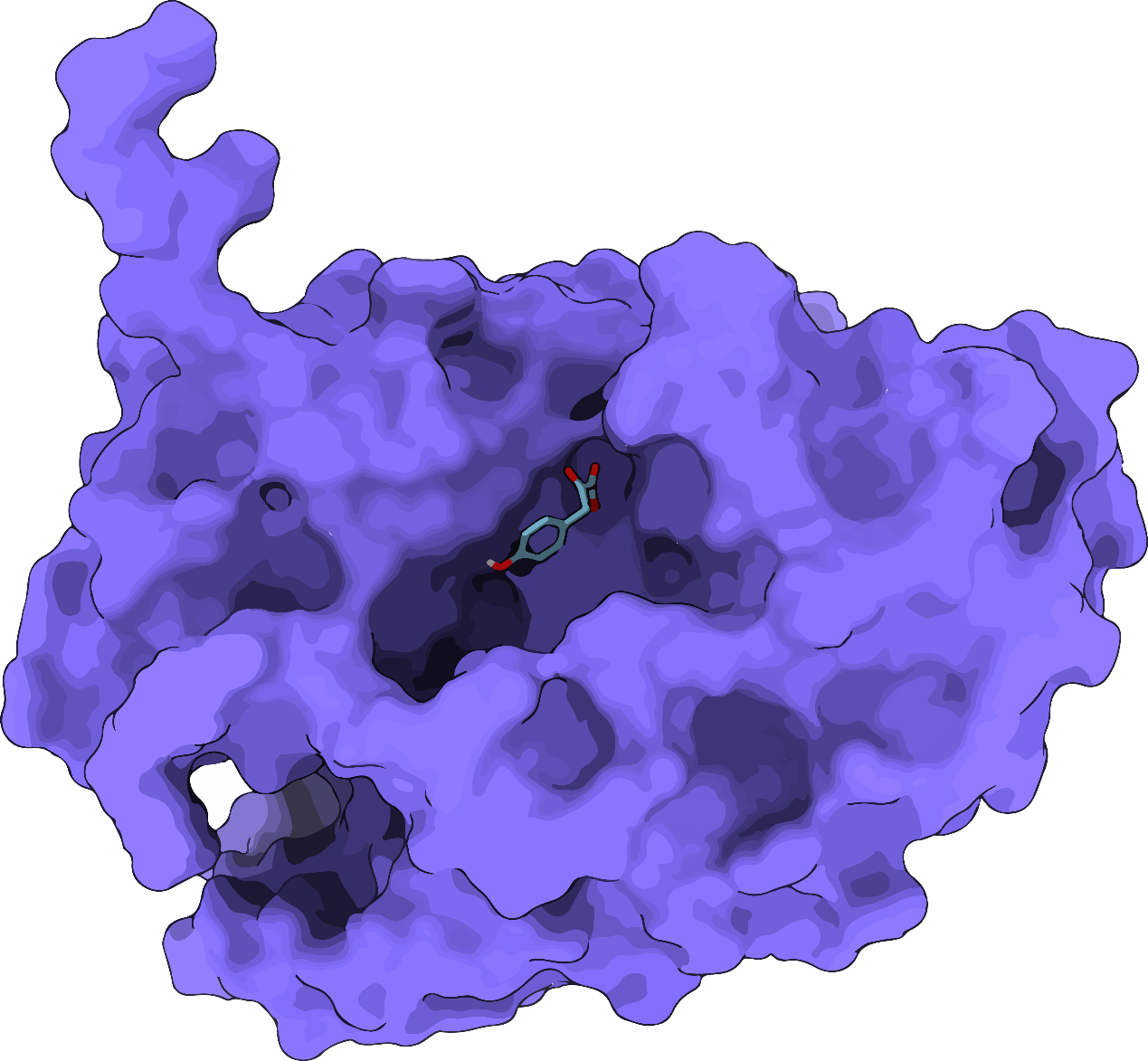